The human immune system plays a vital role in protecting the body from infection, harmful substances, and cellular changes such as cancer.
Boosting the immune system to treat cancers was first described in the late 19th century,1 when Dr. William B. Coley discovered that soft tissue and bone sarcomas could be controlled in some patients by injecting them directly with heat-inactivated bacteria. (These later became known as “Coley’s toxins.”2)
More recent cancer treatment approaches have utilized immune response mediators such as interleukin and targeted vaccines but with limited success.
A more effective approach developed during the past 20 years has been to enhance the ability of immune cells and in particular T-lymphocytes or T-cells, to recognize, target, and eliminate tumor cells. Two main pathways have emerged: immune checkpoint inhibitors, which work by activating T-cells; and CAR T-cell therapy, which modifies and enhances T-cell receptor function.
The immunotherapy path contrasts with chemotherapy and biological or targeted therapies, which work by attacking vital cell functions to kill cancer cells. There is increasing recognition of the importance of the tumor microenvironment for the development and progression of cancer. The side effect profile of immunotherapy is different from that of chemo or targeted therapies, so may offer a better quality of life to individuals with advanced cancers undergoing such treatments.
This article reviews how modern immunotherapy is transforming cancer outcomes, and the real possibility that it might even cure some highly lethal cancers such as malignant melanoma.
Immune System
The two main components of the immune system are the innate and adaptive systems. Both work together to protect the body against attack from harmful agents.
The innate immune system is composed of dendritic cells and macrophages, i.e., antigen presenting cells. These cells patrol the body and engulf any foreign antigens or proteins associated with pathogens and cancer cells. These are then presented to T-cells (T lymphocytes), which are the main component of the adaptive immune system.
T-cells are a type of white blood cell. They originate from stem cells in the bone marrow and mature in the thymus into one of three types of cells: helper T-cells, cytotoxic T-cells, and regulatory T-cells. These cells can reside in the peripheral tissues or circulate in the blood or lymphatic system. Based on the nature of the glycoprotein on the cell surface, cytotoxic T-cells are also known as CD8+ cells and helper T-cells as CD4+ cells.18
Helper T-cell activation triggers a cascade that allows the immune system to eradicate cells carrying a foreign antigen. They are involved in stimulating cytotoxic T-cells to proliferate and attack and in stimulating B lymphocytes (B-cells) to proliferate and mature into plasma cells which produce antibodies.19
Regulatory T cells (also known as TREGs) can suppress the immune response, and thus have a critical role in preventing autoimmunity. Their function is to inhibit T-cell proliferation and production of cytokines.
The adaptive immune system recognizes and remembers specific pathogens to create long-lasting immunity. T-cells carry unique receptors for detecting specific antigens.
Biology-Immune Checkpoint Inhibitors
To prevent autoimmunity, i.e., an attack by a person’s own immune cells on their autoantigens, the human immune system has several key checkpoints that switch off T-cell responses. In a healthy body, this inhibitory regulation of immune cells can also minimize collateral tissue damage by controlling the duration and extent of an immune response.
Certain cancer cells can survive and continue to proliferate by harnessing immune checkpoint pathways in order to evade detection and destruction by the immune system. Earlier immunotherapy drugs targeted immune checkpoint proteins PD-1 and CTLA-4 on T-cells, and modern approaches to cancer immunotherapy use antibodies that, in essence, blockade specific immune checkpoint molecules.
Figure 1 shows the programmed cell death (PD) pathway. (Programmed cell death, or apoptosis, is a normal physiological process.) The receptor on the surface of the T-cell is called PD-1. The ligand (or protein) that binds to this receptor is called PD-L1 (programmed death ligand 1). PD-L1 may be present on both cancerous and normal cells.
In certain cancers, when a T-cell’s PD-1 receptor connects with a cancer cell’s PD-L1 ligand, the T-cell is “switched off,” which means it will not attack the tumor cell despite being able to detect it. It is as if the cancer cell has become invisible to the T-cell. Immune checkpoint inhibitor therapies work by breaking that immune checkpoint pathway, either by blocking the PD-1 receptor or the PD-L1 ligand, thereby allowing T-cells to become active.
Figure 1:
The T-Cell Tumor Blockade
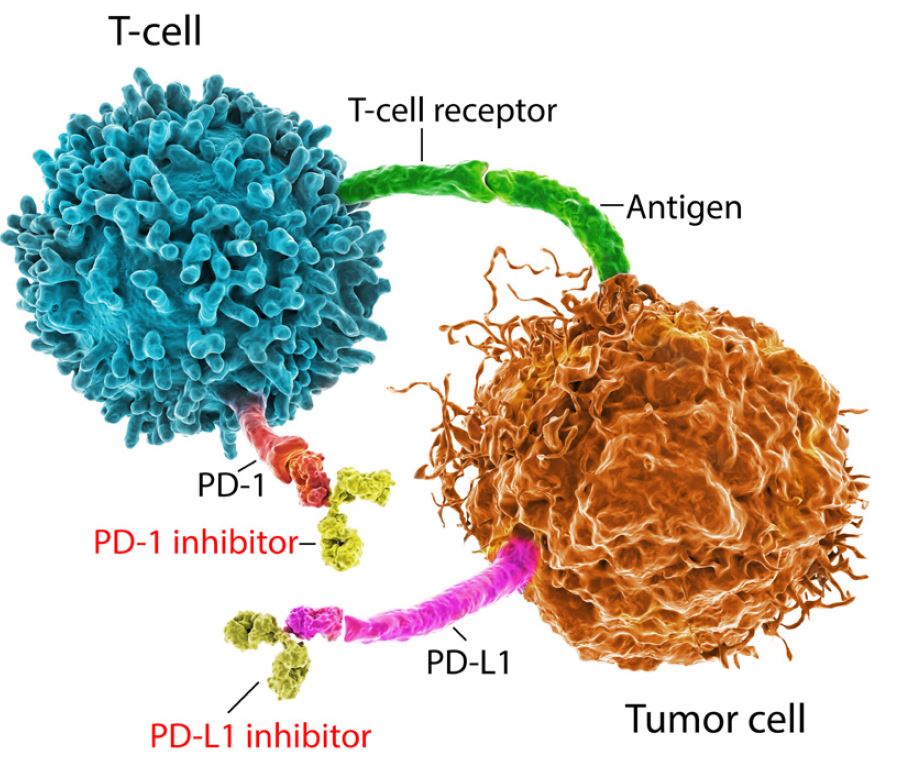
Source: Adobe Stock
Immune Checkpoint Inhibitors in Malignant Melanoma
Immune checkpoint inhibitor therapies act as blocks to cancer cell proliferation. They have revolutionized treatment of a range of cancers; in particular malignant melanoma.
Metastatic melanoma is a highly lethal cancer with a five-year survival rate of no better than 20% to 30%.3 Melanomas in visceral sites such as the liver and the brain have the worst outlook, with average life expectancies of less than one year.4
Recent trials of certain immune checkpoint inhibitors are yielding interesting results. The CheckMate-067 clinical trial, for example, has been studying the anti-PD-1 drug nivolumab and the anti-CTLA-4 drug ipilimumab as potential treatments for advanced malignant melanoma in previously untreated patients. The trial recruited 945 patients with stage 3 (spread to lymph nodes) and stage 4 (spread to distant sites) cancers. Participants were grouped into three arms: one received nivolumab and ipilimumab combination therapy; one received only nivolumab; and one received only ipilimumab. Patients in each of the arms received four courses of treatment followed by maintenance nivolumab until they experienced either disease progression or intolerable adverse events.
As shown in Table 1, nearly two-thirds of the patients receiving nivolumab had ongoing responses five years after starting treatment, whether given with ipilimumab or on its own. About a fifth of this patient cohort also experienced a complete response (remission), with no sign of cancer on scans after completing treatment.
Many of those receiving combination therapy also experienced long and sustained control of their cancers, with median survival rates of more than five years after starting treatment. These are remarkable results from a well-conducted clinical trial and suggest it may be possible to cure some advanced melanomas.
One downside of immune checkpoint inhibitor therapy is that adverse events are common. The reason for this is that by targeting a checkpoint protein, T-cells are switched on and may attack cells throughout the body, including skin, bowel, liver, lung, kidney, and endocrine glands. Although adverse events can be moderate to severe (grade 3 and grade 4), they are rarely life-threatening and usually resolve within 12 weeks of starting treatment.
Table 1: Results of CheckMate 067 Trial20 |
---|
| Nivolumab + Ipilimumab | Nivolumab alone | Ipilimumab alone |
Ongoing Response at Five Years | 62% | 61% | 40% |
Complete Response Rate | 22% | 19% | 6% |
Median Survival (months) | >60 | 36.9 | 19.9 |
Adverse Events – Grades 3 (moderate severe) and 4 (severe) | 59% | 23% | 28% |
Immune Checkpoint Inhibitors' Efficacy
In the last ten years, immunotherapies have transformed outcomes for many patients with advanced stage cancers. This has not only been for malignant melanomas but also for lung, head and neck, breast, renal, and bladder cancers. In fact, many stage 4 cancer patients who received immunotherapy treatments have achieved durable responses and significantly improved life expectancies.21
One drawback of immunotherapy, however, is that many patients do not respond to it. Indeed, only an estimated 12% of all cancer patients will benefit from immune checkpoint inhibitor treatment.5 Still, several new immunotherapy drugs are under development and clinical trials are planned for a wide range of cancer types.
Although side effects of checkpoint inhibitor drugs, which can include constipation/diarrhea, fatigue, cough, nausea, muscle/joint pain, and more, are not comfortable, they are better tolerated than those of chemotherapy. Still, there is the potential for the side effects to be severe, resulting in poorer quality of life and even fatality. Considerable effort is currently underway to identify predictive biomarkers that will indicate who may respond well to these treatments and for whom they may have little to no benefit.
Clinical experience also suggests that factors such as tumor mutational burden, mismatch repair deficiency, and PD-L1 expression levels may be able to indicate potential treatment efficacy. At the moment, however, there are no reliable biomarkers for selecting patients for treatment.6 That being said, obtaining PD-L1 expression levels is recommended in the treatment guidelines for non-small-cell lung cancer.
Tumor Mutational Burden
The tumor mutational burden (TMB) is the number of mutations in the DNA of a cancer cell.22 Cancer is characterized by the continuous proliferation of cells. This generally begins with genetic mutations that occur randomly during the process of DNA replication for cell division. Genetic mutations have the potential to produce abnormal proteins, which may be expressed by cells as cancer antigens. The higher the number of mutations, the higher the likelihood of cells generating antigens which can dysregulate immune checkpoints on the T-cells. This hypothesis has been supported by an analysis of more than 1,600 patients with a range of cancers including malignant melanoma, non-small-cell lung cancer, and bladder cancer.
Higher tumor mutational burden has been associated with longer survival and better response rates to immune checkpoint inhibitor therapies. However, there does not appear to be a common predictive cutoff point for all cancer types, which means the clinical utility of predicting response to therapy is uncertain.7
Mismatch Repair Deficiency
The mismatch repair pathway plays a key role in identifying and repairing mismatched bases during DNA replication. The repair process avoids insertions or deletions of abnormal DNA in microsatellites (short repeated sequences of DNA). Defects or deficiencies in this process, known as deficient mismatch repair (dMMR), result in high levels of microsatellite instability (MSI-H) and accumulation of DNA mutations within the cell. Tumors with dMMR are referred to as having high TMB.
Defects of MMR genes may be due to germline mutations, as seen in certain hereditary cancers such as Lynch syndrome, or can occur spontaneously. Patients with Lynch syndrome are susceptible to developing multiple cancers, especially colon and endometrial cancer. dMMR occurs in about 15% of sporadic colon cancer.8
There are currently numerous clinical trials of pembrolizumab, an immunotherapy drug that targets PD-1 in a range of advanced dMMR cancers which have become resistant to chemotherapy. Overall, about 40% of patients in these trials had some benefit from immunotherapy treatment. The responses lasted more than six months in 78% of patients, with a range from 1.6 to 27 months.9 These results led to the U.S. FDA approving pembrolizumab for the treatment of inoperable or metastatic dMMR/MSI-H cancers as a second line or subsequent therapy irrespective of the site or type of cancer.
PD-L1 Expression
PD-1/PD-L1 checkpoints have important functions in maintaining immune tolerance. These checkpoints are also hijacked by cancer cells to avoid detection and destruction by the immune system. In metastatic non-small-cell lung cancer, immune checkpoint inhibitors using either a PD-1 or a PD-L1 blockade are now embedded in best-practice therapy guidelines, as clinical trials have shown a doubling of median survival rates with this immunotherapy.
The Keynote series of clinical trials focus on pembrolizumab as an immune checkpoint inhibitor and are being conducted for a wide range of cancers, with notable results to date. The Keynote-024 clinical trial, which is testing pembrolizumab in cancers with high expression of PD-L1 (at least 50%), found that the therapy nearly doubled median survival to 26.3 months from 13.4 months for chemotherapy.10
The Keynote-189 clinical trial, which focused on cancer patients expressing intermediate levels of PD-L1 (1-49%), showed that adding pembrolizumab to chemotherapy in advanced metastatic non-small-cell lung cancer improved the one-year survival rate from 49% to 69%. Survival at two years also increased, rising from 27% to 46%.11
Despite the utility of PD-L1 as a biomarker for guiding the selection of treatment for non-small-cell lung cancer, a review of studies that supported FDA approval of immune checkpoint inhibitors in a range of cancers highlighted the lack of consistency in methodology, such as the type of assay used, type of cell studied (tumor, immune, or both), and expression of cutoff values for defining the level of expression. The review also reported that positive PD-L1 values predicted improved response to immunotherapy in less than 30% of studies.12
CAR T-cell Therapy
CAR T-cells are another type of immunotherapy-based cancer treatment. CAR, or chimeric antigen receptor, is a synthetic protein engineered for T-cells that enable them to detect a specific antigen on a cancer cell.
Figure 2 shows the multi-week laboratory and clinical process for providing autologous CAR-T therapy. T-cells from a patient’s blood are isolated using apheresis, a process where cells are separated from plasma. The T-cells are then engineered into CAR-T cells by inserting a gene into them that codes for CAR. After allowing the resulting CAR T-cells to multiply, they are infused back into the patient.
CAR T-cell therapy has been referred to as a “living drug,” as these engineered T-cells are stable, continue to multiply in the body, and can detect and eradicate cancer cells if they reappear later in time. It is planned as a one-off treatment with long-lasting effects.
The U.S. FDA and the U.K.’s National Institute of Clinical Excellence (NICE) have both given approval for using CAR T-cell therapy for B-cell acute lymphoblastic leukemia (ALL) for those up to the age of 25 years and for adults with three types of blood cancer: diffuse large B-cell lymphoma; mediastinal B-cell lymphoma; and mantle cell lymphoma. Treatment can be given when the blood cancer is either refractory to first-line treatment or has relapsed after at least two lines of therapy.
Figure 2:
Process for Autologous CAR-T Therapy
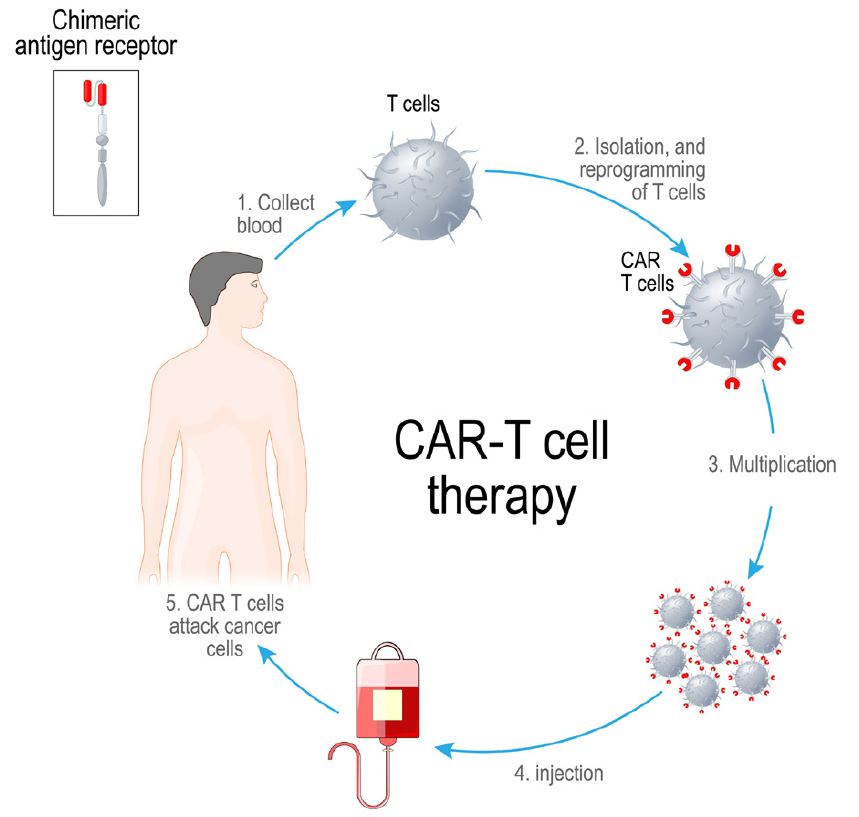
Source: Adobe Stock
CAR T-cell therapy was first approved by the FDA for clinical use in 2017. Early clinical reports indicated a complete response rate of greater than 80%,13 and some patients who have had this treatment achieved sustained remission of more than three years.14 Longer-term follow-up is being undertaken to define more precisely the duration of response and to see whether patients can in fact be cured.
Clinical indications for CAR T-cell therapy are blood cancers, as its use for solid tumors is limited by factors such as the solid tumor microenvironments, which prevent CAR T-cells from reaching target cancer cells, antigen expression by both cancerous and normal cells, and variable expression of antigens within a specific patient, which may even be absent in many cells.15